Biomedical engineers at the University of Melbourne have fabricated artificial vascular grafts that could prove useful during essential bypass surgery.
Bypass surgery saves lives but is by no means simple. Conventional methods involve autologous grafts or those made of synthetic material; however, these may not always be a viable alternative for the vessel in question, or a patient’s comorbidities may prohibit a graft using native blood vessels from another part of the body.
A new study from a team of biomedical engineers at the University of Melbourne presents a possible new solution – artificially engineered vascular grafts.
Mimicking blood vessels
Crafting functional blood vessels is a deceptively challenging undertaking, Associate Professor Daniel Heath, ARC Future Fellow at the University of Melbourne’s Department of Biomedical Engineering, told create.
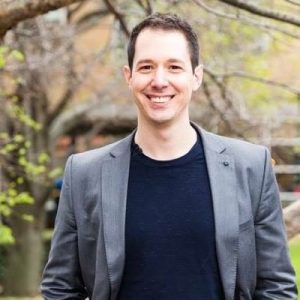
“Blood vessels are complex, multi-layered structures,” he said. “An inner layer of endothelial cells with anticoagulant abilities is responsible for keeping blood from clotting inside the body. A vessel must also have the appropriate mechanical properties; it must be strong enough to support the blood pressure without rupture or aneurysm.
“So blood vessels have a layer of circumferentially aligned smooth muscle cells that responds to changes in blood pressure and provide mechanical strength.”
The endothelial cells align in the direction of blood flow, Heath explained, and the smooth muscle cells form rings around the vessel.
Fabricating an effective substitute to these cells in a laboratory setting, and particularly in a fast, cheap and scalable way, was a challenge Heath and his team were committed to solving.
They first used a process called electrospinning to craft thin polymer fibres that would form the basis of the engineered vessel.
“We spun the fibre on a mandrel to create a tube,” Heath explained. “These fibres provide mechanical strength to our blood vessel mimic to prevent it from rupturing, and also allow it to be sutured to an existing blood vessel for implantation.”
There was a catch, however: the fibres they deposited were randomly oriented.
“When we put endothelial cells on the surface of the polymer frame, they didn’t align in the direction of the blood vessel – and this alignment is critical to their function,” he said.
To solve the issue, the electrospun polymer tube was placed into a rigid container, filled with water and frozen.
“Since the container was rigid, the ice crystals were forced to grow in a longitudinal direction, which reorganised the fibres axially.”
Troubleshooting
The next step involved applying smooth muscle cells to bolster the vessel’s mechanical strength.
“We cast a layer of hydrogel around the polymer layer, allowing us to support a three-dimensional structure of muscle cells, and then looked at different stiffnesses for the hydrogel layer,” Heath said.
“We found that at an intermediate concentration, the cells spontaneously oriented circumferentially, much like a native blood vessel. And truthfully, we’re not exactly sure why.”
This response varied to that of the cells at higher and lower concentrations of stiffness, Heath explained, when the cells did not spontaneously align.
“There’s a sweet spot where the smooth muscle cells start to behave like they should in vivo, aligning and forming rings around the vessel.”
Heath said that expensive bioreactors are usually necessary to achieve the appropriate cellular morphology, which is made easier and more accessible with electrospinning.
“Using bioreactors, it can take weeks for the vessel to mature appropriately and have the right structure,” he said. “With the electrospinning shortcut we can make it happen much faster and in a more scalable manner.”
Lab to clinic
Heath said both of the core innovations occurred “without too much head-scratching”.
“It’s very rare in research, but very fortuitous for us,” he said. “The idea is that the vascular grafts will be remodelled over time. The current base materials will degrade, so eventually we want to replace that with a fully functional, tissue-based engineered blood vessel.
“The main limitation is that studies can be very expensive. Often you need investor support to champion a technology in order to bridge the gap between what’s found in the lab and what actually gets to the clinic.
“Translating these concepts into clinical application would save a lot of lives, extend lifespans and reduce the burden of disease. That’s the ultimate goal – but there are a lot of steps that need to happen between now and then.”
Read how another biomedical engineer is helping us better understand the bloodstream – and fight heart disease.
Image: http://www.scientificanimations.com, CC BY-SA 4.0 <https://creativecommons.org/licenses/by-sa/4.0>, via Wikimedia Commons