Researchers thought engineering super-smooth materials would stop bacteria attaching to surfaces. They were wrong.
When Distinguished Professor Elena Ivanova was researching anti-microbial materials a decade ago, it was believed bacteria wouldn’t be able to stick to smooth surfaces.
She started studying mirror-like surfaces, looking even at atomically-smooth materials to see if they could repel microorganisms. The results were deflating, to say the least.
“Initially, people thought that if we make a surface very, very smooth … bacteria will not be able to attach on the surface,” Ivanova told create. “To our huge surprise, bacteria actually liked that.
“They managed to attach nicely and develop biofilms on the nanoscopically smooth surfaces. And that was so disappointing.”
Ivanova, a nanobiotechnologist who leads the Mechano-biocidal Materials Research Group at RMIT University, looked to nature for inspiration.
She started with lotus leaves, which are famous for being water-repellent and ‘self-cleaning’. But it was in insect wings that she discovered something remarkable.
Looking at cicada and grasshopper wings with powerful microscopes, the researchers saw they were covered in tiny nanopillars.
These spiky features were about 200 to 400 nanometres tall. About 250 nanopillars stacked on top of each other would be roughly the width of a human hair.
Ivanova said bacterial cells were able to settle on the surface of the wings, but they didn’t look healthy. Her team realised the spikes were rupturing the bacteria.
“It was clear that bacteria [were] killed on the surfaces of insect wings,” Ivanova said. “That was really the start of [this] work.”
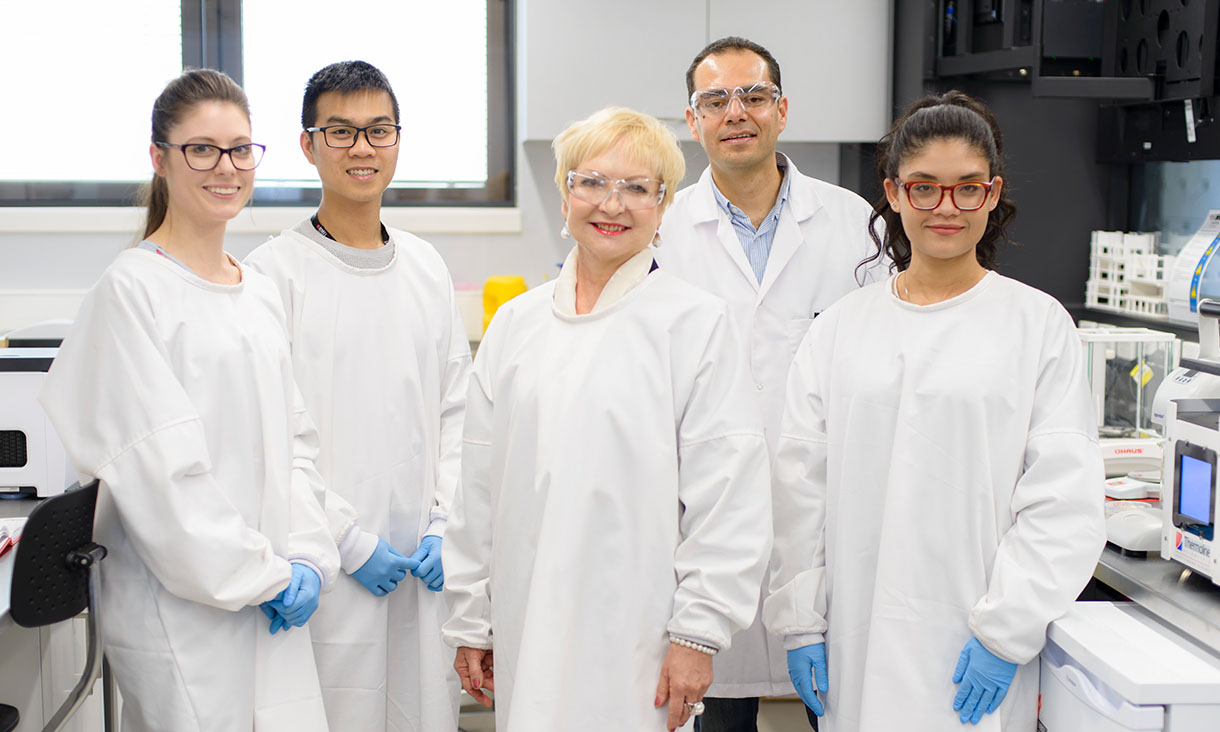
Micro-spikes vs drug-resistant fungi
Ivanova and her team began experimenting with techniques to recreate the features they saw in nature on different materials.
The group recently published a study on what happens when multi drug-resistant Candida fungus lands on titanium covered with micro-spikes.
The fungus is a potentially deadly microorganism responsible for one in 10 hospital-acquired medical device infections.
Ivanova found the spikes consistently killed about half of the Candida cells that landed on the surface. But even more exciting for the researchers was the fate of the other half of the cells.
Even though they were only injured, they were ultimately unable to survive, even when the researchers tried to revive them under better conditions.
“Injured Candida cells were unable to progress with their development of the biofilms,” Ivanova said. “And eventually, that injury triggered a programmed cell death.”
Killing 100 per cent of the microorganisms is important to avoid them developing resistance, in the same way many bacteria have become resistant to antibiotics.
“It’s extremely important to understand that, actually, injured cells will not survive,” Ivanova said. “That was really very exciting.”
To avoid infection around implants such as titanium hips or dental prosthesis, doctors typically use a range of antimicrobial coatings, chemicals and antibiotics.
But these fail to stop antibiotic-resistant strains and can even increase resistance.
Instead, micro-spikes could be used on implants to combat infections from drug-resistant superbugs.
Ivanova said it was important that the engineered materials act against a range of different bugs.
“The surface will be working against different types of microorganisms, not only against bacteria … but also against Candida,” she explained.
“That involves different sizes, different metabolic activity, different construction of the cells. And it’s very difficult to have a universal pattern, which will [work] for all types of bacteria.”
From insects to aeroplanes
Ivanova’s team created the micro-spike pattern in titanium with a technique called plasma etching, a method that could be optimised for a wide range of materials and applications.
“The beauty of nanotechnology and nanofabrication is that once you know the pattern works … you can reproduce the same pattern in different types of materials,” Ivanova said. “It could be stainless steel, it could be plastic, it could be glass.”
The team is now working to commercialise the technology, collaborating with a dental school to produce dental implants featuring the anti-microbial spikes.
Ivanova also sees applications in other fields, such as in aerospace. She said nanostructures could improve the surfaces of aircraft, or be used in filters to help purify the air in-flight.
After ten years, Ivanova believes there’s still a lot to learn about nanostructures and the features engineers can create on surfaces.
She said different nanofeatures — ranging from sharp points to pillars — can kill bacteria in different ways. Both hydrophobic and hydrophilic surfaces can work — it all comes down to the nanopattern.
The group is learning more and more about remarkable surfaces created in nature.
“I still think it’s the tip of the iceberg, even after ten years,” she said. “We’re talking about 3.6 [to] 3.7 billion years of evolution. [Bacteria] can adapt to any environment or any surface. That’s something you can fight only looking back to nature.”