The promise of additive manufacturing can run aground when it comes to applying the technique at scale. That’s why Mark Helou is getting creative.
As additive manufacturing has become better understood, more affordable and more usable, an increasing number of businesses are taking up the process.
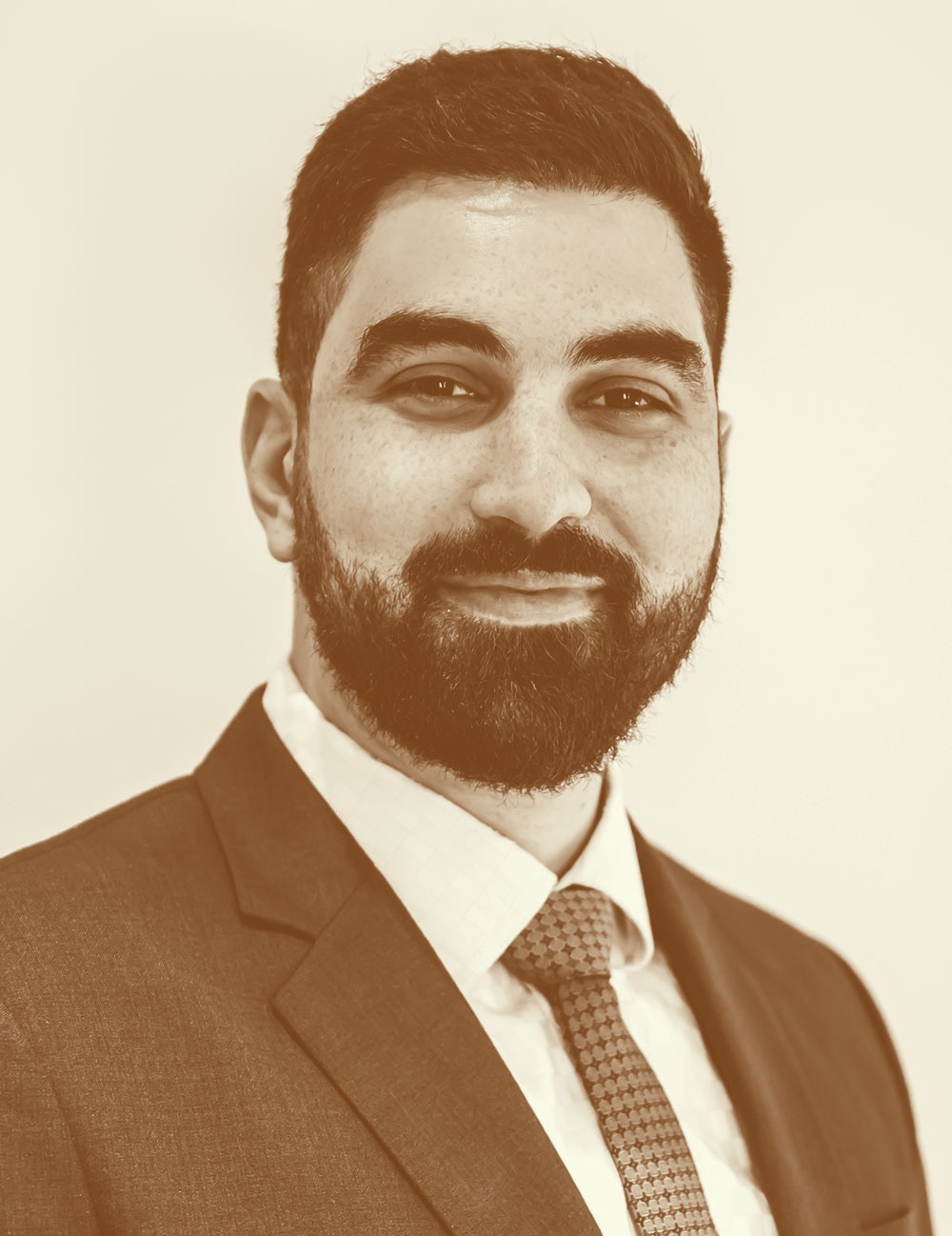
“But the machines are getting to a point where you can’t really optimise the manufacturing too much more,” Mark Helou, Research and Development and Business Development Manager at Nepean Engineering and Innovation, told create.
“You can add more lasers and increase the bed space, but that comes with a new set of problems. The only way to really increase the speed of manufacturing is [to have] less material there.”
The speed issue has rendered additive manufacturing unsuitable for most volume production. One solution comes in the form of lattice structures, a reasonably new field of research and the focus of Helou’s current PhD work.
These space-filling cells have also been used to lower material and energy use — both of which represent non-trivial costs — and increase the strength-to-weight ratio of printed parts.
To highlight the current cost of printing, Helou points to an experiment considered early in his doctoral research. Requiring 3000 titanium specimens, it would have cost nearly a million dollars, according to his estimate.
The variables for a cell can include dimensions, strut diameter and orientation.
Uniform and random
Though there are countless types of lattice, they broadly fit into two families: periodic and stochastic.
The former is made of uniform cells, each geometrically defined. The latter is randomised, with the dimensions of cells unable to be predicted accurately — for example the arrangement on the inside of a cork.
Some CAD companies allow users to design their own lattices, but do not recommend which types might best suit a given application. These recommendations are the focus of Helou’s research.
“[I have reviewed] three different lattice structure types with different thicknesses of struts, with different amounts of trusses in a given envelope … testing that boundary and getting data points so I can then extrapolate the information without testing each single component,” he explained.
“That way I can search in that boundary space to choose the right lattice structure. And then if that works you can increase that design space and do more testing and do more lattice structures and more sizes and different materials — like titanium and cobalt chrome and stainless steel.
“Then very quickly you have this growing amount of data sets which you can extrapolate information from and look at certain parameters which you haven’t tested, but you can with a high certainty of confidence know how it will perform.”
Having a better set of options to choose from, rather than a selection that might seem to be infinite, has obvious appeal.
The right structure for the right application is a tricky thing to guess at, adds Helou, with multiple objectives to satisfy.
“For a femoral implant, for example, you might need a lot of compressive strength,” he said, adding that this case would need to resist compression in the right way, but also withstand impact from the side, perpendicular to the main axis.
“So, you have to optimise for both of those.”
Bones and cars
Lattices for light-weighting aerospace parts, for example, might have a broadly familiar structure.
But besides saving in weight and cost, printed lattices have also gained interest for their potential to absorb energy, shock and noise.
Research has included filling voids with liquids and gases, allowing the structure to wick away heat, and injecting polymers to increase energy absorption properties.
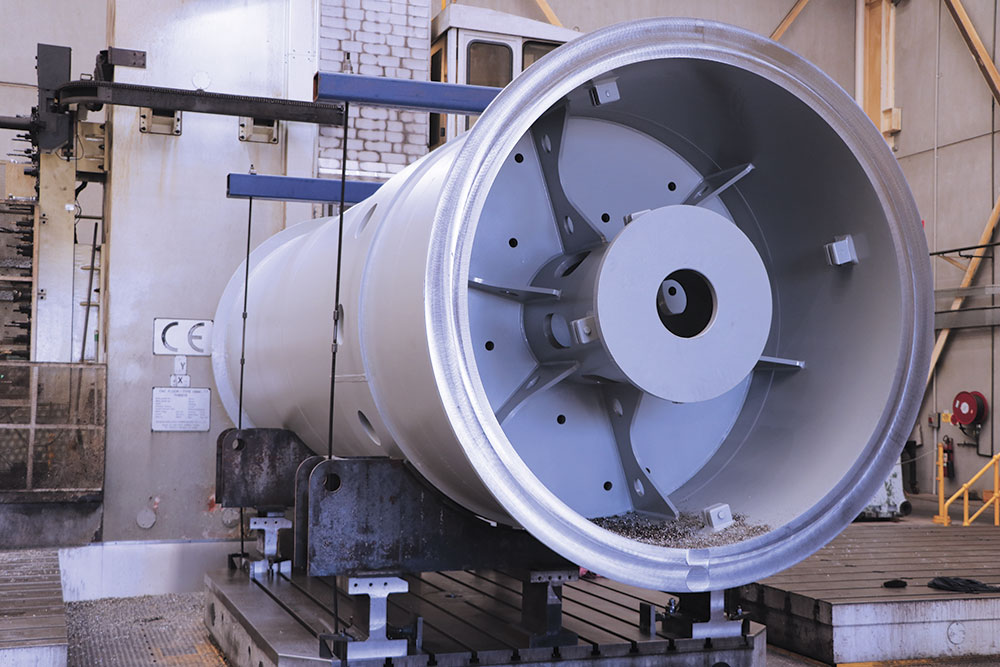
Lattice structures could also enable some products, such as automotive parts, to be more easily recycled. Helou has investigated this application as part of Professor Sami Kara’s Sustainable Manufacturing and Life Cycle Engineering Research Group at the University of New South Wales.
A mono-material chassis, for example, could be tailored to have different strength and stiffness at different points by varying the size of lattices, but could also be easily melted down for reuse when the vehicle was discarded.
Another potential automotive application involves “multiple yield point” properties, which he explored in his honour’s thesis, where a layer of cells collapses, and a sacrificial part increases in strength.
“It forms more of a solid, and what happens is it dissipates the energy better,” he explained.
“An example is within the crumple zone of a car; when the car crashes, it dissipates the energy, but actually it’s becoming more and more solid and becoming stronger and stronger.”
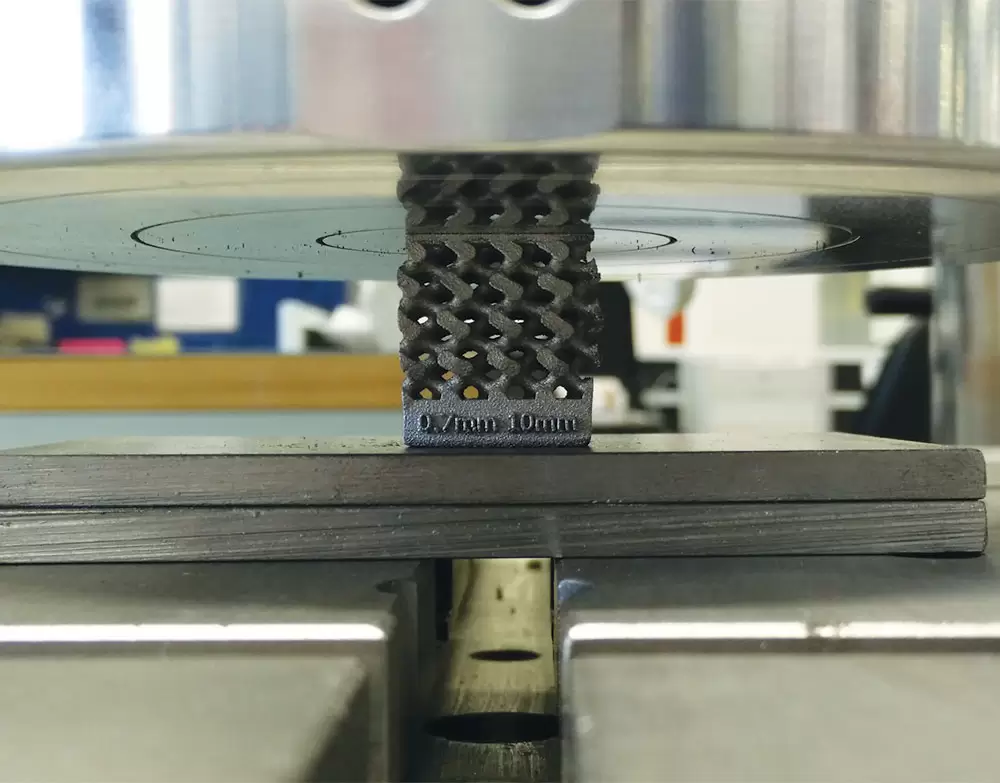
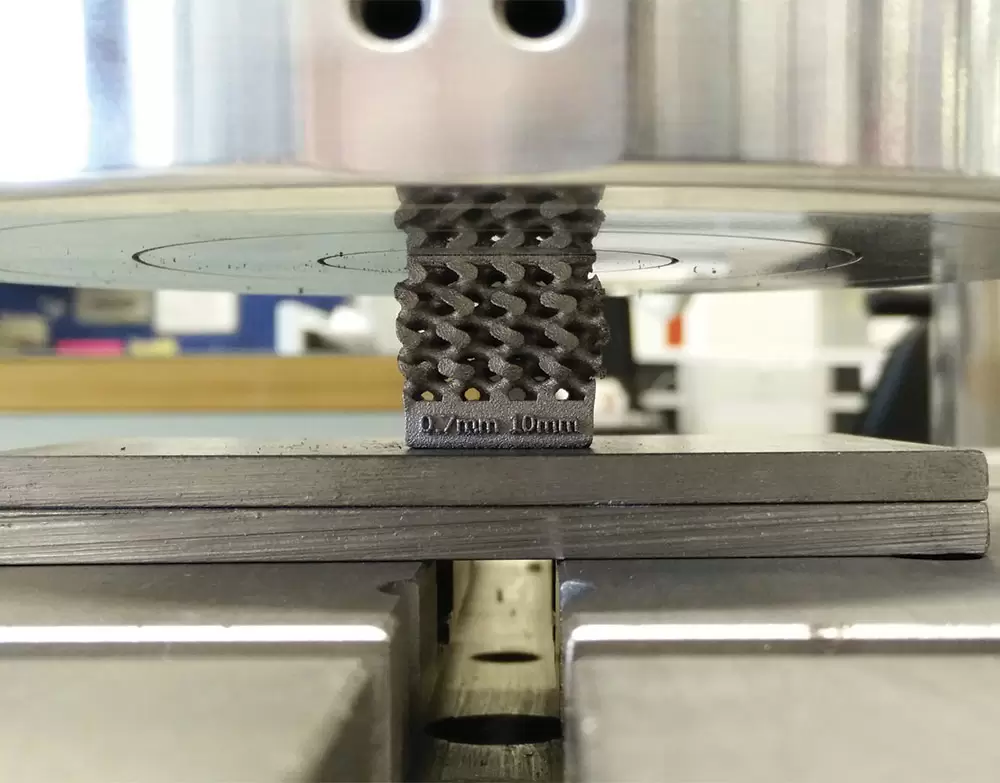
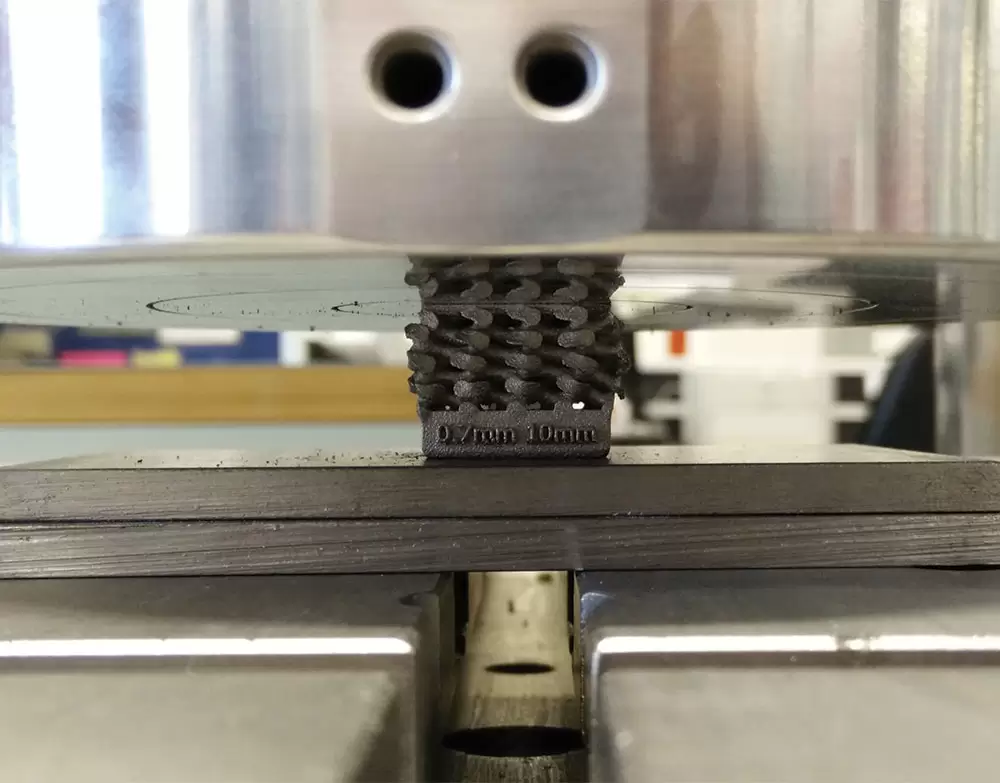
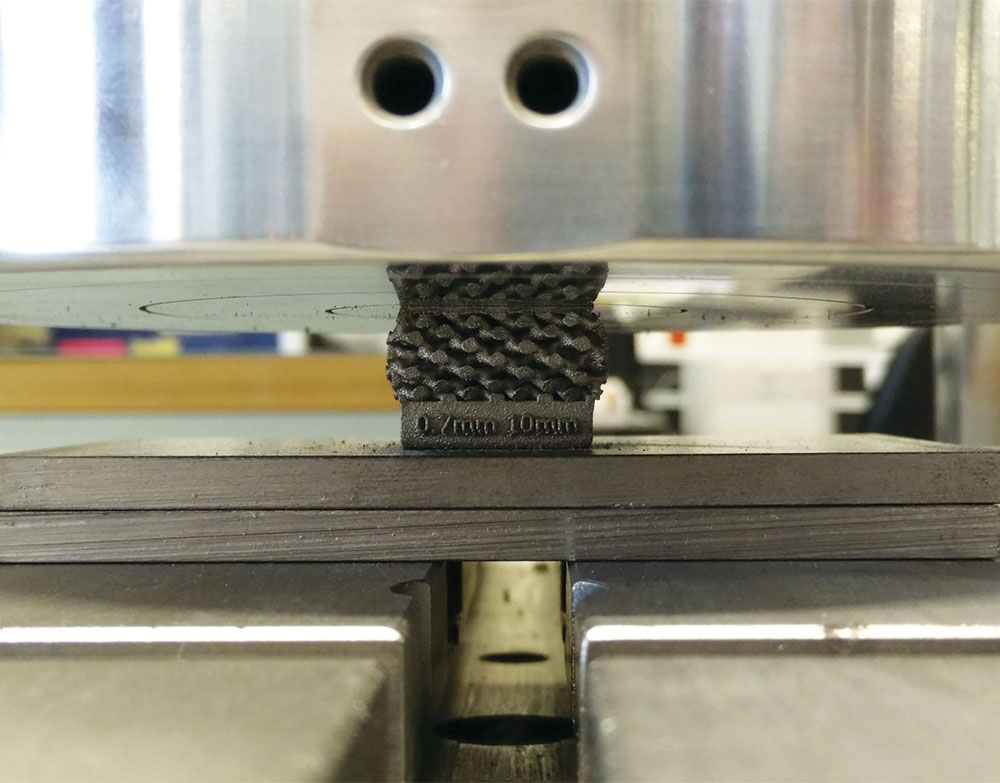
Hands-on approach
Helou came to 3D printing by accident. While studying for his mechatronics degree at the University of New South Wales, Helou worked as a research and development engineer at BresMedical, whose parent company Breseight is a pioneer adopter of 3D printing in Australia.
“The experience I gained there in additive manufacturing was unheard of,” he recalled.
“It’s different to use additive manufacturing in the capacity of industry versus academia.
“I learned so much hands-on … I didn’t like the theoretical side too much. I liked being hands-on and designing stuff and solving problems.”
Helou began his current role at Nepean in October 2020 and is helping to modernise manufacturing at the country’s biggest privately owned engineering firm.
Shortly before create spoke to him, the company applied for a NSW government grant to support investment in an industrial-grade 3D printer.
Helou was also preparing his final experiment and planning his dissertation.
His short to medium-term plans involve bringing Nepean, a half-century old manufacturer into a new era, rather than focusing on details at the cell-level.
“We have identified so many different places where the company could go,” he said.
“Because the company is so vast and broad, we could go anywhere. But we have to be very selective in what you do, because R&D is not free. So, you have to choose where you want to go.”
Manufacturing the future
Helou heads a research and development team of one at Nepean, the foundation division of a multi-divisional, family-owned company dating back to 1974.
Nepean currently has no products of its own; when these are developed, they are spun off into other parts of the company. This spans 1500 employees in eight countries, earning revenues of about $500 million annually.
Each month, about 550 new jobs all covering multiple industries come in to the company’s headquarters in Narellan, New South Wales.
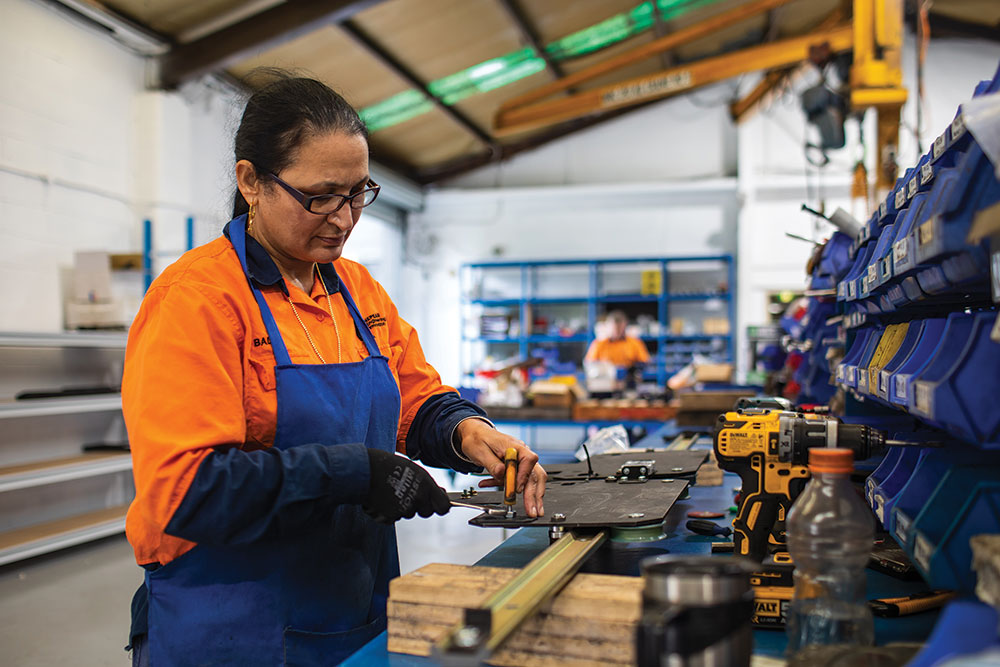
On site are machinery investments worth about $50 million, with some of this approaching end of life. Upwards of 2000 tonnes of steel per job are regularly provided for construction industry customers.
“I was brought on to start to transition the company into something a bit newer and more agile,” Helou explained, adding that medical technology — which is a big part of his background — was a growth opportunity.
When create visited the Nepean facility, the company was preparing for a stage two audit to gain ISO 13485 certification, covering QMS for medical devices.
Investments in additive manufacturing machines are also likely to be made.
Once a workforce is familiar with and skilled enough in its use to practically apply additive manufacturing, it can be transformative for a company, Helou said.
“Having the machinery here and getting your head around how it works — it’s just invaluable.”